SOLAR COOLING OVERVIEW
1. INTRODUCTION
Cooling buildings and products accounts for more than 20% of the electrical energy demand of an urban city (Waite et al., 2017) and can reach up to 62% of the peak daily electrical demand in cities with high active cooling penetration (Ali et al., 2011; Waite et al., 2017). Solar energy can be utilized to sustainably meet much of our space air-conditioning and refrigeration needs due to its accessibility, scalability, and availability as compared to other renewable energy resources, such as wind, geothermal, and hydro (Li et al., 2015). A wide range of cooling technologies presented in Fig. 1 can be powered by solar energy.
![Cooling technologies that can be coupled with solar harvesting technologies [adapted from Kimand Infante Ferreira (2008) and Alazazmeh and Mokheimer (2015)]](/content/10200/fig1.png)
Figure 1. Cooling technologies that can be coupled with solar harvesting technologies [adapted from Kimand Infante Ferreira (2008) and Alazazmeh and Mokheimer (2015)]
Cooling technologies can be broadly categorized based on their driving mechanisms as electrically driven technologies, which include mechanically powered technologies and thermally driven technologies, where the major energy input is in the form of heat. Hybrid cooling refers to cooling cycles incorporating a combination of the cooling technologies coupled together in either a serial or parallel fashion to improve overall system performance, increase operating temperature range, and achieve better humidity control (Gado et al., 2021). System performance improvement can be derived from utilization of waste heat or minimization of the temperature differential, referred to as lift. Leveraging the desiccant sorption principle in hybrid cooling can enable efficient humidity control.
Cooling technology performance is represented by the coefficient of performance (COP), which is defined as units of cooling derived from each unit of electrical and/or thermal energy input. The working principle, COP range, merits, and limitations of cooling technologies are given in Table 1.
TABLE 1: Cooling technologies working principle, COP, merits, and limitations
Name | Working Principle | COP | Merits | Limitations | Refs. |
---|---|---|---|---|---|
Vapor compression | A condensable gas with high latent heat capacity is mechanically compressed, condensed, and then expanded to achieve cooling effect. | 2–6 |
|
|
Otanicar et al. (2012) |
Stirling | An enclosed gas in two chambers separated by a porous and high thermal capacity material is mechanically compressed and expanded to produce cooling effect. | 0.8–3 |
|
|
Daoud (2019) |
Thermoacoustic | Acoustic vibrations are generated in a cavity with an enclosed gas to develop successive compression and expansion gas regions, which are amplified through use of a low thermal conductivity porous material resulting in cooling. | 0.8–1.7 |
|
|
Poese et al. (2004), Raut and Wankhede (2017) |
Magnetic | Fluctuating magnetic fields cause temperature change within a paramagnetic material which in conjunction with a heat transfer fluid is used to deliver the cooling effect. | 1.8–3.5 |
|
|
Greco et al. (2019), Zhang et al. (2021) |
Thermoelectric/ Peltier | A voltage potential difference applied to dissimilar semiconductors results in a temperature differential resulting in cooling. | 0.1–0.6 |
|
|
Sarbu and Dorca (2018) |
Electrochemical | Reversible electrochemical reactions in two fuel cells can result in a temperature differential with the difference between charging and discharging voltage provided by a voltage source. | 0.9–2.5 |
|
|
Abdollahipour and Sayyaadi (2021) |
Absorption | A liquid mixture comprised of chemically absorbed vapor is pressurized through liquid pumping, which is then heated to release vapor that is condensed and expanded to achieve cooling. In an open cycle, air is dehumidified by passing through a spray of liquid sorbent that is regenerated through heating. | 0.1–2.0 |
|
|
Mokhtar et al. (2010), Ghafoor and Munir (2015), Xu and Wang (2016) |
Adsorption | A gas is physically adsorbed on a solid sorbent, which is heated to release pressurized gas that is condensed and expanded to achieve cooling. The cycle is maintained using at least two adsorption chambers, which alternate between functioning as an adsorber and desorber. In an open cycle, air is dehumidified through passing through a solid sorbent wheel that is regenerated through flow of unsaturated air. | 0.5–0.7 |
|
|
Ghafoor and Munir (2015) |
Ejector | Same as vapor compression except that compression is done using a dual inlet and single outlet converging-diverging nozzle in which heated gas provides the motive force for cooled vapor to mix with the heated gas and increase pressure in the diverging part of the nozzle. | 0.1–0.6 |
|
|
Besagni et al. (2016) |
Sky radiation | Selective emission of the solar spectrum between 8 and 13 µm wavelengths using special surface coatings is used to radiate heat to the environment during daytime and nighttime resulting in cooling. | — |
|
|
Zhao et al. (2019) |
2. REPRESENTATIVE SOLAR COOLING TECHNOLOGIES
The majority of the cooling technologies presented in Table 1 are at the research stage. Commercialization has been achieved for vapor compression, absorption, adsorption, and ejector cooling technologies. In solar electrical, vapor compression cooling is the most widely deployed technology particularly at small scale (Köll and Neyer, 2018) due to its high performance, while absorption cooling has a > 70% market share in solar thermal cooling (Sparber et al., 2009). Desiccants have a long (conventional) history of use in space air-conditioning systems to achieve a desired humidity level, referred to as desiccant cooling (Kim and Infante Ferreira, 2008). One representative configuration of both of these technologies coupled with solar harvesting technologies is presented in Fig. 2. Information on the characteristics of a few selected solar thermal cooling systems deployed worldwide is presented in Table 2.
![]() | ![]() |
(a) | (b) |
Figure 2. (a) Schematic of a representative solar electrical cooling system: PV panel coupled vapor compression cycle and (b) schematic of a representative solar thermal cooling system: Non-concentrating collector coupled single-effect absorption cycle [adapted from Ghafoor and Munir (2015)]
TABLE 2: Representative small-scale (< 100 kW) solar cooling plants installed worldwide, based on Neyer et al. (2018)
Location | Solar Cooling Technology | Cooling Capacity (kW) | COP | Energy Storage |
---|---|---|---|---|
Garching, Germany | PV-vapor compression chiller | 22.4 | 4.1 | No battery storage but latent heat storage |
Hurghada, Egypt | PV-vapor compression chiller | 6 | 2.6 | 2.4 kWh battery storage |
Mikkel, Finland | Evacuated tube collector-single effect absorption chiller | 1024 (Air-cooler) | 0.7 | 2000 L water storage |
Palermo, Italy | Linear Fresnel collector-double effect absorption chiller | 23 (Integrated cooling tower) | 1.1 | 400 kWh molten salt thermocline storage |
Photovoltaics (PV) coupled vapor compression cooling has gained traction in recent years primarily due to decreased PV prices and because vapor compression cooling systems can achieve a COP well above 3 (Otanicar et al., 2012). A representative configuration of solar electrical cooling is shown in Fig. 2(a).
As another alternative, Fig. 2(b) depicts a schematic of absorption cooling—a technology that can be coupled with solar thermal technologies. Absorption cooling represents one of the most common thermally driven cooling processes. It generally employs aqueous mixtures, such as LiBr-H2O and NH3-H2O, that absorb water in the cooled state and are regenerated later in the process by (solar) heating in the regenerator (Hassan and Mohamad, 2012; Labus et al., 2013).
Absorption cooling systems can increase their performance through the inclusion of multiple regenerator-condenser pairs or regenerator-absorber pairs referred to as “effects,” but this comes at the cost of higher required driving temperatures (Xu and Wang, 2016). If higher driving temperatures are provided by solar collectors, it will reduce the thermal efficiency of the collectors due to thermal losses, as noted in Fig. 3(a). On the basis of COP, several thermal-driven cooling options are compared in Fig. 3(b), which indicates that a linear increase in performance is possible with higher driving temperatures. Pulling these two efficiency trends together, it can be inferred that the challenge for solar thermal cooling is to optimize the driving temperature to maximize overall system efficiency.
![]() | ![]() |
(a) | (b) |
Figure 3. (a) Typical non-concentrating collector efficiency variation with deriving temperatures at 800 W/m2 and 25°C ambient (Reprinted with permission from Henning, Copyright 2000) and (b) Thermal cooling systems performance variation with driving temperatures (Reprinted from Balaras et al. with permission from Elsevier, Copyright 2007)
Representative combinations of the abovementioned cooling technologies with different solar harvesting technologies are presented in Fig. 4, containing typical collector efficiency, driving temperature, and COP of cooling technologies. A comparative analysis of these combinations for a hot climate by Mokhtar et al. (2010) revealed that solar electrical cooling employing vapor compression energetically outperforms other solar cooling combinations. The analysis compared solar cooling technologies using overall system efficiency defined as cooling output obtained for a given solar input over land area sized to achieve a solar fraction of 75%. System efficiency of solar electrical cooling was estimated to be more than four times greater than the single effect absorption cycle with non-concentrating collectors, followed by concentrating collectors combined with a high-performance thermal cooling technology (such as triple-effect absorption cooling technology). Solar thermal cooling based on absorption/adsorption cooling is generally utilized commercially for medium to large size (> 100 kW) cooling capacity systems with up to a 1750 kW cooling capacity flat-plate, single-effect absorption chiller system installed in 2014 in Arizona (Weiss and Spörk-Dür, 2021).
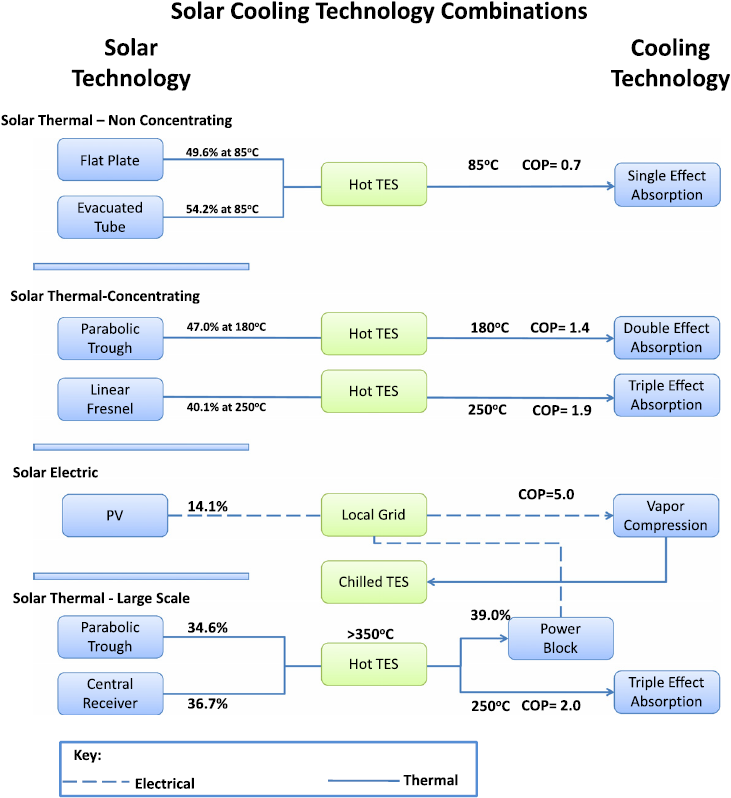
Figure 4. Typical solar cooling combinations and their yearly average performance for a hot climate (Reprinted from Mokhtar et al. with permission from Elsevier, Copyright 2010)
3. ENERGY STORAGE REQUIREMENT
Coupling cooling technologies with an intermittent renewable resource is best done with some amount of energy storage to ensure the energy supply is available to meet the cooling demand, as shown in Fig. 5(a) for hot-dry and warm-humid climates categorized according to the Köppen–Geiger climate classification (Beck et al., 2018). Energy storage at small-to-medium scale can be done via chemical battery storage for electrical cooling technologies or via hot water for thermal cooling technologies. Large-scale solar systems can make use of sensible solid storage, such as concrete (with a heat transfer fluid) or liquid storage (e.g., molten salts). Seasonal storage can greatly increase reliability of using solar resources for powering thermal cooling systems, especially in hot climates, as shown in Fig. 5(b). However, the development of cost-effective seasonal energy storage systems is currently an active area of research (Xu et al., 2014; Yang et al., 2021).
![]() (a) |
![]() (b) |
Figure 5. (a) Typical daily cooling load and solar resource availability profiles for hot and humid climates adapted from Infante Ferreira and Kim (2014) and Pintaldi et al. (2017) and (b) yearly profile of electricity demand and solar resource components for hot climate (Reprinted from Mokhtar et al. with permission from Elsevier, Copyright 2010)
4. CONCLUSION
Solar cooling (with appropriate energy storage) represents a sustainable approach toward meeting the building and product cooling demand. As was mentioned previously, a wide range of solar cooling technologies is available, but two technologies stand out: (i) PV electric-driven vapor compression and (ii) solar thermal-driven absorption systems. It is suggested that, as a first pass evaluation, overall system efficiency should be considered when comparing solar cooling systems. Using this metric shows that electrically driven vapor compression systems are currently the most efficient way of meeting cooling needs for small-to-medium–scale systems. Overall, it is expected that, as these technologies become more efficient and cost-effective, they will start to play a central role in our society's cooling technology portfolio.
REFERENCES
Abdollahipour, A. and Sayyaadi, H. (2021) A Review of Thermally Regenerative Electrochemical Systems for Power Generation and Refrigeration Applications, Appl. Therm. Eng., 187: 116576.
Alazazmeh, A. and Mokheimer, E. (2015) Review of Solar Cooling Technologies, J. Appl. Mech. Eng., 4(5): 180–194.
Ali, M.T., Mokhtar, M., Chiesa, M., and Armstrong, P. (2011) A Cooling Change-Point Model of Community-Aggregate Electrical Load, Energy Build., 43(1): 28–37.
Balaras, C.A., Grossman, G., Henning, H.-M., Infante Ferreira, C.A., Podesser, E., Wang, L., and Wiemken, E. (2007) Solar Air Conditioning in Europe—An Overview, Renew. Sustain. Energy Rev., 11(2): 299–314.
Beck, H.E., Zimmermann, N.E., McVicar, T.R., Vergopolan, N., Berg A., and Wood, E.F. (2018) Present and Future Köppen–Geiger Climate Classification Maps at 1-km Resolution, Sci. Data, 5(1): 180214.
Besagni, G., Mereu, R., and Inzoli, F. (2016) Ejector Refrigeration: A Comprehensive Review, Renew. Sustain. Energy Rev., 53: 373–407.
Daoud, J.F.M.S. (2019) Development of a Solar Powered Refrigeration System: Stirling-Cycle Based, PhD, University of Edinburgh.
Gado, M.G., Ookawara, S., Nada, S., and El-Sharkawy, I.I. (2021) Hybrid Sorption-Vapor Compression Cooling Systems: A Comprehensive Overview, Renew. Sustain. Energy Rev., 143: 110912.
Ghafoor, A. and Munir, A. (2015) Worldwide Overview of Solar Thermal Cooling Technologies, Renew. Sustain. Energy Rev., 43: 763–774.
Greco, A., Aprea, C., Maiorino, A., and Masselli, C. (2019) A Review of the State of the Art of Solid-State Caloric Cooling Processes at Room-Temperature before 2019, Int. J. Refrig., 106: 66–88.
Hassan, H.Z. and Mohamad, A.A. (2012) A Review on Solar Cold Production through Absorption Technology, Renew. Sustain. Energy Rev., 16(7): 5331–5348.
Henning, M. (2000) Air-Conditioning with Solar Energy, SERVITEC Meeting, Barcelona.
Infante Ferreira, C. and Kim, D.-S. (2014) Techno-Economic Review of Solar Cooling Technologies Based on Location-Specific Data, Int. J. Refrig., 39, pp. 23–37.
Kim, D.S. and Infante Ferreira, C.A. (2008) Solar Refrigeration Options – A State-of-the-Art Review, Int. J. Refrig., 31(1): 3–15.
Köll, R. and Neyer, D. (2018) Monitoring Data Analysis On Technical Issues & On Performances, IEA SHC Task 53, Gleisdorf, Austria: AEE – Inst. for Sustainable Technol. and Univ. of Innsbruck, p.101.
Labus, J.M., Bruno, C.J., and Coronas, A. (2013) Review on Absorption Technology with Emphasis on Small Capacity Absorption Machines, Therm. Sci., 17(3): 739–762.
Li, K., Bian, H., Liu, C., Zhang, D., and Yang, Y. (2015) Comparison of Geothermal with Solar and Wind Power Generation Systems, Renew. Sustain. Energy Rev., 42: 1464–1474.
Mokhtar, M., Ali, M.T., Bräuniger, S., Afshari, A., Sgouridis, S., Armstrong, P., and Chiesa, M. (2010) Systematic Comprehensive Techno-Economic Assessment of Solar Cooling Technologies Using Location-Specific Climate Data, Appl. Energy, 87(12): 3766–3778.
Neyer, D., Köll, R., and Quiles, P.G.V. (2018) Catalogue of Selected Systems, IEA SHC Task 53. Feldgasse 19, 8200 Gleisdorf, Austria, AEE – Institute for Sustainable Technologies, University of Innsbruck and Miguel Hernández University of Elche, p. 20.
Otanicar, T., Taylor, R.A., and Phelan, P.E. (2012) Prospects for Solar Cooling – An Economic and Environmental Assessment, Sol. Energy, 86(5): 1287–1299.
Pintaldi, S., Sethuvenkatraman, S., White, S., and Rosengarten, G. (2017) Energetic Evaluation of Thermal Energy Storage Options for High Efficiency Solar Cooling Systems, Appl. Energy, 188: 160–177.
Poese, M.E., Robert, W.M., and Garret, S.L. (2004) Thermoacoustic Refrigeration for Ice Cream Sales, 6th IIR Gustav Lorentzen Conference on Natural Working Fluids, Glasgow, UK: Int. Inst. of Refrigeration.
Raut, A.S. and Wankhede, U.S. (2017) Review of Investigations in Eco-Friendly Thermoacoustic Refrigeration System, Therm. Sci., 21(3): 1335–1347.
Sarbu, I. and Dorca, A. (2018) A Comprehensive Review of Solar Thermoelectric Cooling Systems, Int. J. Energy Res., 42(2): 395–415.
Sparber, W., Napolitano, A., Eckert, G., and Preisler, A. (2009) State of the Art on Existing Solar Heating and Cooling Systems–Technical Report Subtask B, IEA SHC Task 38.
Waite, M., Cohen, E., Torbey, H., Piccirilli, M., Tian, Y., and Modi, V. (2017) Global Trends in Urban Electricity Demands for Cooling and Heating, Energy, 127: 786–802.
Weiss, W. and Spörk-Dür, M. (2021) Solar Heat Worldwide, Global Market Development and Trends, 8200 Gleisdorf, Austria, AEE – Institute for Sustainable Technologies, pp. 1–86.
Xu, J., Wang, R.Z., and Li, Y. (2014) A Review of Available Technologies for Seasonal Thermal Energy Storage, Sol. Energy, 103: 610–638.
Xu, Z.Y. and Wang, R.Z. (2016) Absorption Refrigeration Cycles: Categorized Based on the Cycle Construction, Int. J. Refrig., 62: 114–136.
Yang, T., Liu, W., Kramer, G.J., and Sun, Q. (2021) Seasonal Thermal Energy Storage: A Techno-Economic Literature Review, Renew. Sustain. Energy Rev., 139: 110732.
Zhang, Y., Wu, J., He, J., Wang, K., and Yu, G. (2021) Solutions to Obstacles in the Commercialization of Room-Temperature Magnetic Refrigeration, Renew. Sustain. Energy Rev., 143: 110933.
Zhao, D., Aili, A., Zhai, Y., Xu, S., Tan, G., Yin, X., and Yang, R. (2019) Radiative Sky Cooling: Fundamental Principles, Materials, and Applications, Appl. Phys. Rev., 6(2): 021306.
References
- Abdollahipour, A. and Sayyaadi, H. (2021) A Review of Thermally Regenerative Electrochemical Systems for Power Generation and Refrigeration Applications, Appl. Therm. Eng., 187: 116576.
- Alazazmeh, A. and Mokheimer, E. (2015) Review of Solar Cooling Technologies, J. Appl. Mech. Eng., 4(5): 180–194.
- Ali, M.T., Mokhtar, M., Chiesa, M., and Armstrong, P. (2011) A Cooling Change-Point Model of Community-Aggregate Electrical Load, Energy Build., 43(1): 28–37.
- Balaras, C.A., Grossman, G., Henning, H.-M., Infante Ferreira, C.A., Podesser, E., Wang, L., and Wiemken, E. (2007) Solar Air Conditioning in Europe—An Overview, Renew. Sustain. Energy Rev., 11(2): 299–314.
- Beck, H.E., Zimmermann, N.E., McVicar, T.R., Vergopolan, N., Berg A., and Wood, E.F. (2018) Present and Future Köppen–Geiger Climate Classification Maps at 1-km Resolution, Sci. Data, 5(1): 180214.
- Besagni, G., Mereu, R., and Inzoli, F. (2016) Ejector Refrigeration: A Comprehensive Review, Renew. Sustain. Energy Rev., 53: 373–407.
- Daoud, J.F.M.S. (2019) Development of a Solar Powered Refrigeration System: Stirling-Cycle Based, PhD, University of Edinburgh.
- Gado, M.G., Ookawara, S., Nada, S., and El-Sharkawy, I.I. (2021) Hybrid Sorption-Vapor Compression Cooling Systems: A Comprehensive Overview, Renew. Sustain. Energy Rev., 143: 110912.
- Ghafoor, A. and Munir, A. (2015) Worldwide Overview of Solar Thermal Cooling Technologies, Renew. Sustain. Energy Rev., 43: 763–774.
- Greco, A., Aprea, C., Maiorino, A., and Masselli, C. (2019) A Review of the State of the Art of Solid-State Caloric Cooling Processes at Room-Temperature before 2019, Int. J. Refrig., 106: 66–88.
- Hassan, H.Z. and Mohamad, A.A. (2012) A Review on Solar Cold Production through Absorption Technology, Renew. Sustain. Energy Rev., 16(7): 5331–5348.
- Henning, M. (2000) Air-Conditioning with Solar Energy, SERVITEC Meeting, Barcelona.
- Infante Ferreira, C. and Kim, D.-S. (2014) Techno-Economic Review of Solar Cooling Technologies Based on Location-Specific Data, Int. J. Refrig., 39, pp. 23–37.
- Kim, D.S. and Infante Ferreira, C.A. (2008) Solar Refrigeration Options – A State-of-the-Art Review, Int. J. Refrig., 31(1): 3–15.
- Köll, R. and Neyer, D. (2018) Monitoring Data Analysis On Technical Issues & On Performances, IEA SHC Task 53, Gleisdorf, Austria: AEE – Inst. for Sustainable Technol. and Univ. of Innsbruck, p.101.
- Labus, J.M., Bruno, C.J., and Coronas, A. (2013) Review on Absorption Technology with Emphasis on Small Capacity Absorption Machines, Therm. Sci., 17(3): 739–762.
- Li, K., Bian, H., Liu, C., Zhang, D., and Yang, Y. (2015) Comparison of Geothermal with Solar and Wind Power Generation Systems, Renew. Sustain. Energy Rev., 42: 1464–1474.
- Mokhtar, M., Ali, M.T., Bräuniger, S., Afshari, A., Sgouridis, S., Armstrong, P., and Chiesa, M. (2010) Systematic Comprehensive Techno-Economic Assessment of Solar Cooling Technologies Using Location-Specific Climate Data, Appl. Energy, 87(12): 3766–3778.
- Neyer, D., Köll, R., and Quiles, P.G.V. (2018) Catalogue of Selected Systems, IEA SHC Task 53. Feldgasse 19, 8200 Gleisdorf, Austria, AEE – Institute for Sustainable Technologies, University of Innsbruck and Miguel Hernández University of Elche, p. 20.
- Otanicar, T., Taylor, R.A., and Phelan, P.E. (2012) Prospects for Solar Cooling – An Economic and Environmental Assessment, Sol. Energy, 86(5): 1287–1299.
- Pintaldi, S., Sethuvenkatraman, S., White, S., and Rosengarten, G. (2017) Energetic Evaluation of Thermal Energy Storage Options for High Efficiency Solar Cooling Systems, Appl. Energy, 188: 160–177.
- Poese, M.E., Robert, W.M., and Garret, S.L. (2004) Thermoacoustic Refrigeration for Ice Cream Sales, 6th IIR Gustav Lorentzen Conference on Natural Working Fluids, Glasgow, UK: Int. Inst. of Refrigeration.
- Raut, A.S. and Wankhede, U.S. (2017) Review of Investigations in Eco-Friendly Thermoacoustic Refrigeration System, Therm. Sci., 21(3): 1335–1347.
- Sarbu, I. and Dorca, A. (2018) A Comprehensive Review of Solar Thermoelectric Cooling Systems, Int. J. Energy Res., 42(2): 395–415.
- Sparber, W., Napolitano, A., Eckert, G., and Preisler, A. (2009) State of the Art on Existing Solar Heating and Cooling Systems–Technical Report Subtask B, IEA SHC Task 38.
- Waite, M., Cohen, E., Torbey, H., Piccirilli, M., Tian, Y., and Modi, V. (2017) Global Trends in Urban Electricity Demands for Cooling and Heating, Energy, 127: 786–802.
- Weiss, W. and Spörk-Dür, M. (2021) Solar Heat Worldwide, Global Market Development and Trends, 8200 Gleisdorf, Austria, AEE – Institute for Sustainable Technologies, pp. 1–86.
- Xu, J., Wang, R.Z., and Li, Y. (2014) A Review of Available Technologies for Seasonal Thermal Energy Storage, Sol. Energy, 103: 610–638.
- Xu, Z.Y. and Wang, R.Z. (2016) Absorption Refrigeration Cycles: Categorized Based on the Cycle Construction, Int. J. Refrig., 62: 114–136.
- Yang, T., Liu, W., Kramer, G.J., and Sun, Q. (2021) Seasonal Thermal Energy Storage: A Techno-Economic Literature Review, Renew. Sustain. Energy Rev., 139: 110732.
- Zhang, Y., Wu, J., He, J., Wang, K., and Yu, G. (2021) Solutions to Obstacles in the Commercialization of Room-Temperature Magnetic Refrigeration, Renew. Sustain. Energy Rev., 143: 110933.