MODELING DROPWISE CONDENSATION OVER TEXTURED SURFACES
Vapor-to-liquid phase-change process in the form of discrete drops over or underneath a cold substrate is referred as dropwise condensation. It is realized when the condensate does not wet the substrate except at locations where well-wetted and uniformly distributed contaminant nuclei exist. The heat transfer coefficient during dropwise condensation is usually an order-of-magnitude larger than for filmwise condensation under comparable temperature differentials. This makes dropwise condensation an attractive mechanism for heat exchange applications. However, wide-spread use of dropwise condensation has not been generally successful. This is because of the intricacies faced in controllability and long-term sustainability of the process on textured substrates. Its dependence on parameters such as nucleation site density, hydrophobicity, thickness of a promoter layer, substrate orientation, degree of sub-cooling, and environmental conditions adds to the difficulty in modeling and design. Understanding the entire chain of drop formation, growth and coalescence, contact angle hysteresis, dynamics of the three-phase contact line, and gravitational instability is a challenge, requiring a large research effort. The complexity of the dropwise condensation phenomenon is also a source of scatter in wall heat flux data. In this context, Prof. Muralidhar with his students and collaborators has developed a time- and space-resolved dropwise condensation model that reproduces the details of the process on multiple scales (Sikarwar et al., 2013). The model has been examined for condensation of water vapor as well as moist air and in the condensation of metallic vapor on vertical and inclined surfaces (Somwanshi et al., 2018) and the condensation of water vapor from moist air (Baghel et al., 2020). It has been validated against experiments involving pure water vapor and moist air in terms of drop sizes, cycle times and the overall condensation rate.
The model proceeds with drop formation at preferred nucleation sites at the nanometric scale. These droplets grow by direct condensation, up to a micron size of the order of the distance between neighboring nucleation sites. Beyond this point, coalescence among neighboring drops takes place and subsequent growth of drops occurs by the combination of direct condensation and coalescence. When a drop reaches a millimetric size, body forces exceed surface tension and contact line forces holding it to the solid surface and the drop departs, sweeping the surface clear and permitting new nucleation sites to become available. Hence, coalescence and sliding droplets re-expose substrate area to provide a continuous source of nucleation sites. Overall, dropwise condensation is a cyclic process in time, as represented in Fig. 1.
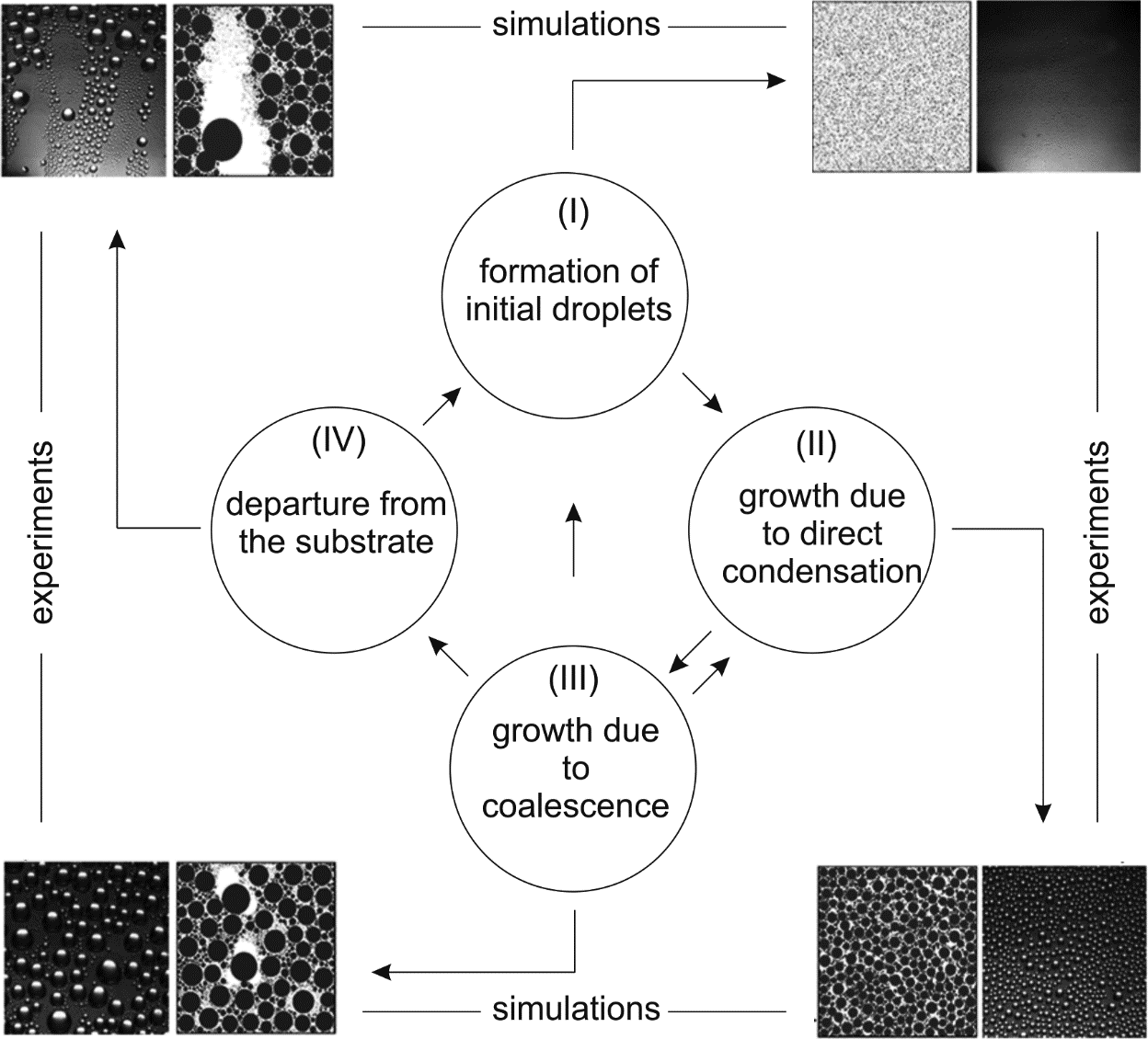
Figure 1. Schematic of the space- and time-resolved dropwise condensation model that proceeds cyclically along steps I–IV, i.e., nucleation to gravitational instability.
Dr. K. Muralidhar, Professor, Department of Mechanical Engineering, Indian Institute of Technology Kanpur (India) has developed a fairly general dropwise condensation model for vapor and vapor-gas mixtures condensing on chemically textured hydrophobic surfaces. The model connects thermodynamics of vapor-liquid phase change on one extreme with surface chemistry, contact line motion, and droplet hydrodynamics on the other. It has the advantage of accepting thermodynamic and thermophysical properties of the gas and vapor phases along with surface details and temperature differentials to initiate the condensation cycle and march forward in time. Since the model is stated in the form of modules, it can be set-up as a computational algorithm and additional details such as wettability gradient and drop deformation can be systematically incorporated (Khandekar and Muralidhar, 2020). The model is also amenable to close validation against experiments at various levels of detail.
REFERENCES
Sikarwar, B.S., Muralidhar, K., and Khandekar, S. (2013) Effect of Drop Shape on Heat Transfer During Dropwise Condensation Underneath Inclined Surfaces, Interfacial Phenomena and Heat Transfer, 1(4), 339–356.
Somwanshi, P., Muralidhar, K., and Khandekar, S. (2018) Dropwise Condensation Patterns of Bismuth Formed on Horizontal and Vertical Surfaces, International Journal of Heat and Mass Transfer, 122, 1024–1039.
Baghel, V., Sikarwar, B.S., and Muralidhar, K. (2020) Dropwise Condensation from Moist Air over a Vertical Hydrophobic Metallic Substrate, Applied Thermal Engineering, 181, 115733.
Khandekar, S. and Muralidhar, K. (2020) Drop Dynamics and Dropwise Condensation on Textured Surfaces, New York: Springer.
Referências
- Sikarwar, B.S., Muralidhar, K., and Khandekar, S. (2013) Effect of Drop Shape on Heat Transfer During Dropwise Condensation Underneath Inclined Surfaces, Interfacial Phenomena and Heat Transfer, 1(4), 339–356.
- Somwanshi, P., Muralidhar, K., and Khandekar, S. (2018) Dropwise Condensation Patterns of Bismuth Formed on Horizontal and Vertical Surfaces, International Journal of Heat and Mass Transfer, 122, 1024–1039.
- Baghel, V., Sikarwar, B.S., and Muralidhar, K. (2020) Dropwise Condensation from Moist Air over a Vertical Hydrophobic Metallic Substrate, Applied Thermal Engineering, 181, 115733.
- Khandekar, S. and Muralidhar, K. (2020) Drop Dynamics and Dropwise Condensation on Textured Surfaces, New York: Springer.