Drops are isolated liquid volumes kept intact by surface tension force. Drops can have sizes ranging from a tenth of a micron to several millimeters. Very small drops, for which gravity force is not significant, and drops in a zero gravity situation have a closely spherical form. The shapes of drops may be changed by external forces.
Drops are formed from jet and sheet splitting, by larger drop breaking down, by drops impacting on a wall and by vapor condensation. Drops are also formed as a result of liquid spraying as gas bubbles burst at interfaces, by splashing, by liquid entrainment by gas flows over interfaces and by boiling liquid passing over a surface with a temperature higher than saturation temperature.
The problem of jet splitting as it flows from an orifice has been examined by Rayleigh. The intensity of jet splitting increases with the dynamic interaction between jet and gas. At some distance from the orifice, unstable waves begin to appear. Along the jet's length, wave amplitude increases as a result of disturbances caused by the relative motion of liquid and gas. Jet splitting takes place when the velocity difference between phases exceeds a critical value (Figure 1a). The amplitudes of short and long waves grow with the difference in velocities. Ryleigh has observed that wave growth rate increases and reaches its maximum at an optimal wavelength λopt, which is roughly 3.5 times the jet diameter. With increasing outflow velocity, the form of fluctuation is changed from symmetric to nonsymmetrical (Figure 1b). The length of unstable waves and drop sizes also decrease.
The sheet from a centrifugal nozzle breaks down with the formation of two groups of waves. The first group of waves moves along the direction of the jet and seeks to transform a sheet into rings. The second group of waves extends to sheet circumference and seeks to separate the liquid in the jet fan spreading out from the nozzle center. As a result of wave motion, drops are formed. The smallest drops are blown away from the film surface under elevated pressures.
Drop flow is affected by inertial, frictional and Archimedean forces. Unlike hard particles, surface deformation and splitting of liquid drops are possible in gas flow. Conditions for the onset of drop splitting can be estimated from stability analysis of spherical interface in flow. Interface stability in the back and front parts of a drop is characterized by the use of the Bond number; instability arises when the Bond number is more than the critical value:
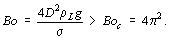
Interface instability in the upper and lower parts of the drop is characterized by the use of the Weber number. Instability occurs when the Weber number is more than the critical value:

With the increasing Weber number, a variety drop splitting mechanisms in a gas flow can be observed, as follows (Figure 2).
Drop splitting to smaller drops takes place at We ≈ Wec;
Drop collapse with preliminary flow of a "bag" formed by a thin liquid film pulled on the ground (splitting of the "parachute" type) is completed at Wec < We < 20, with sufficient time for drop flow and with low viscous liquid. The film breaks down into small drops and on the ground breaks down into big drops;
Drop splitting with formation of a "bag" ("parachute") and jet occurs at 20 < We < 30;
Drop splitting with formation of "bags" and fibres drowned on the flow takes place in the Weber number range of 30 to 60;
Drop splitting resulting in the liquid film or liquid fibres collection being blown away from the drop surface occurs when the Weber number ranges from 60 to 1000;
Explosive splitting is realized at larger Weber numbers. At very high flow velocities, the drop breaks down at once into numerous small drops.
If there is no drop slipping relative to flow, splitting can occur as a result of resonant interaction between the free fluctuation of the drop and a small-scale turbulent vortex, with the drops having close sizes.
If the surface has a temperature near the saturation level and is coated by a liquid film, the impact of drops causes splitting of liquid. If the surface which the drop comes into contact with has a small size, spraying and cutting of drops are possible. If the surface temperature is higher than the temperature at which contact of liquid with the wall is terminated, also called the Leidenfrost temperature (see Leidenfrost Phenomena), a continuous vapor film appears between the drop and the wall. At low We numbers, the drop can recoil from the wall coated by a vapor film and be split. At large We numbers, the drop can spread over the vapor film and break down into numerous small drops.
In drop flow, coagulation, evaporation and condensation of drops can take place.
The coagulation (or coalescence) of drops can take place when drops collide with each other. The result of drop collision is not unique and depends on many factors. At the moment of collision, drops can break down or coalesce. A gas or vapor layer can be conserved between the drops that are close to each other. With increasing drop velocity, the thickness of the gas layer decreases and the probability of collision increases. At some velocity range, drop coagulation takes place.
The vapor-drop system is unstable: drops which have sizes less than a critical value evaporate and disappear; drops which have sizes more than critical value grow as a result of condensation on their surfaces. The critical drop radius rc is coupled to vapor supercooling Ts − TG by the following equation:

where σ is the surface tension; Ts, the saturation temperature; hLG, the latent heat of evaporation; and ρL, the liquid density. If there are a great number of drops with sizes more than critical value in volume, vigorous condensation on drops takes place. The noncondensable gas or vapor mixture leads to the concentration and partial pressure gradients near the drops; this causes a change in the saturation temperature of the diffusive vapor layer. With noncondensable gas, the growth velocity of drops is less than that for condensation of pure vapor because of thermal and partial pressure gradients. Condensation and evaporation velocities increase when gas flows around the drops.
The time of full drop evaporation td depends on the heated surface's temperature Tw. The dependence of water drop evaporation time td, in a volume 5 10-8 m³ placed on a heated surface, on temperature is shown on Figure 3. Three dependences can be distinctly found. Drop evaporation time decreases with an increase of Tw at a small wall superheated relative to saturation temperature. Then drop evaporation time increases sharply in the narrow range of temperature Tw and at last, the value td is reduced again with the high wall temperature. The first downward branch of dependence td(Tw) corresponds to drop boiling; the second upward branch corresponds to the transition to a spheroidal condition, at which the drop is separated from the wall by vapor film; the third, again downward, branch corresponds to a developed spheroidal condition. The function td(Tw) presents approximately the dependence q(ΔT) under condition of pool boiling of liquid.